Li-Ion batteries have packed more energy per gram for a long time compared to Li-Po batteries. The problem for UAV applications has been that Li-Ion can’t deliver enough current, something that is starting to change. Now there are cells that are supposed to be able to deliver 30-35A continuously in the 18650 series, at least according to the specs. Therefor we thought it was time to do some testing and decided to build a 1 cell Li-Ion drone using the Crazyflie Bolt as base.
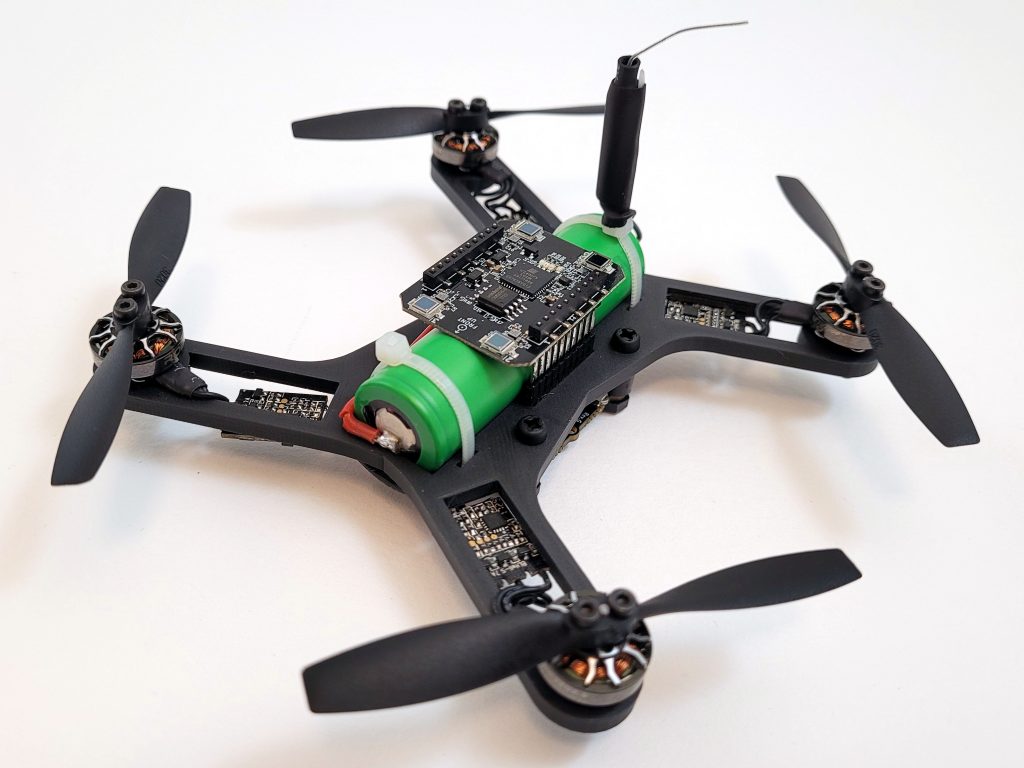
Since a 18650 battery is 18mm in diameter and 65mm long, the size would affect the design but we still wanted to keep the drone small and lightweight. The battery is below 20mm wide which means we can run the deck connectors around it, that is nice. We chose to use our 3D printer to build the frame and use off the shelf ESCs, motors and props. After a couple of hours of research we selected 3″ propellers, 1202.5 11500kv motors and tiny 1-2s single ESCs for our first prototype.
Parts list:
- 1 x Custom designed 130mm 3D printed frame
- 1 x Crazyflie Bolt flight controller
- 4 x Eachine 3020 propeller (2xCW + 2xCCW)
- 4 x Flywoo ROBO RB 1202.5 11500 Kv motors
- 4 x Flash hobby 7A 1-2S ESC
- 1 x Li-Ion Sony 18650 VTC6 3000mAh 30A
- Screws, anti vib. spacers, zipties, etc.
The custom designed frame was developed in iterations, and can still be much improved, but at this stage it is small, lightweight and rigid enough. We wanted the battery to be as central as possible while keeping it all compact.
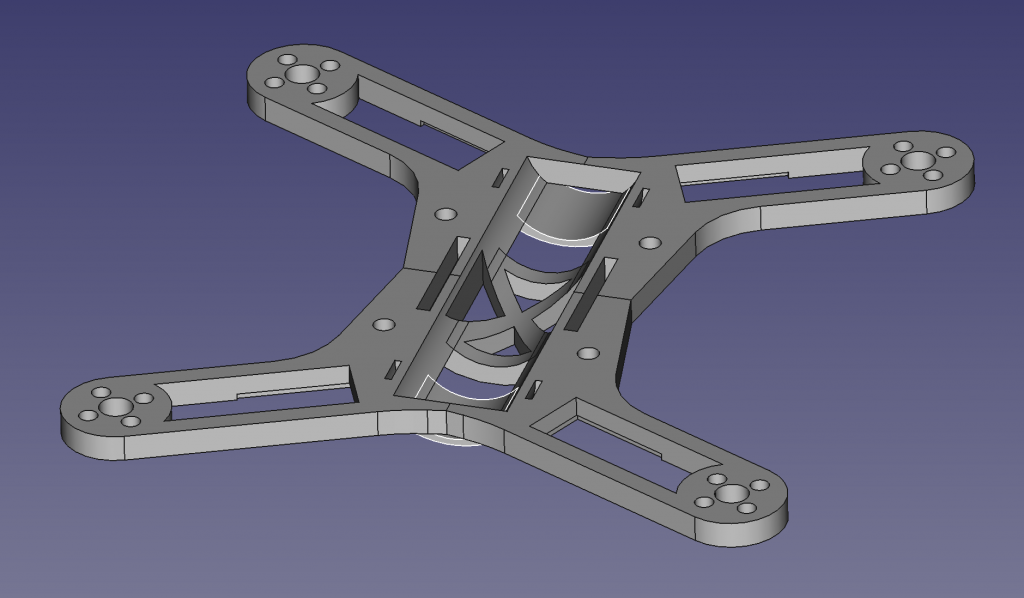
Assembly and tuning
The 3D printed frame came out quite well and weighed in at 13g. After soldering the bolt connectors to the ESCs, attaching motors and props, adjusting battery cable and soldering a XT30 to the Li-Ion battery it all weighed ~103g and then the battery is 45g of these. It feels quite heavy compared to the Crazyflie 2.1 and we had a lot of respect when we test flew it the first time. Before we took off we reduced the pitch and roll PID gains to roughly half and luckily it flew without problems and quite nicely. Well it sounds a lot but that is kind of expected. After increasing the gains a bit we felt quite pleased with:
#define PID_ROLL_RATE_KP 70.0 #define PID_ROLL_RATE_KI 200.0 #define PID_ROLL_RATE_KD 2 #define PID_ROLL_RATE_INTEGRATION_LIMIT 33.3 #define PID_PITCH_RATE_KP 70.0 #define PID_PITCH_RATE_KI 200.0 #define PID_PITCH_RATE_KD 2 #define PID_PITCH_RATE_INTEGRATION_LIMIT 33.3 #define PID_ROLL_KP 7.0 #define PID_ROLL_KI 3.0 #define PID_ROLL_KD 0.0 #define PID_ROLL_INTEGRATION_LIMIT 20.0 #define PID_PITCH_KP 7.0 #define PID_PITCH_KI 3.0 #define PID_PITCH_KD 0.0 #define PID_PITCH_INTEGRATION_LIMIT 20.0
This would be good enough for what we really wanted to try, the endurance with a Li-Ion battery. A quick measurement of the current consumption at hover, 5.8A, we estimated up to ~30 min flight time on a 3000mAh Li-Ion battery, wow, but first a real test…
Hover test
For the hover test we used lighthouse 2 which is starting to work quite well. We had to change the weight and thrust constants in estimator_kalman.c for the autonomous flight to work:
#define CRAZYFLIE_WEIGHT_grams (100.0f) //thrust is thrust mapped for 65536 <==> 250 GRAMS! #define CONTROL_TO_ACC (GRAVITY_MAGNITUDE*250.0f/CRAZYFLIE_WEIGHT_grams/65536.0f)
After doing that and creating a hover script that hovers at 0.5m height and was set to land when the voltage reached 3.0V. We leaned back with excitement, behind a safety net, and started the script… after 19 min it landed… good but not what we hoped for and quite far from the calculated 30 min. Maybe Li-Ion isn’t that good when it needs to provide more current…? A quick internet search and we could find that Li-Ion can run all the way down to 2.5V, but we have to stop at 3.0V because of electronics and loosing thrust, so we are missing quite a bit of energy… Further investigations are needed.
Lighthouse 2 flight test
As a final test we launched some flight scripts to fly in a square and in a spiral so we would get a feel for Lighthouse 2 + Bolt with PID controller combination. We think it turned out quite nicely, and this with almost no optimization effort:
Summary
Li-Ion felt like it could be a game changer when it comes to flight time but was not as promising as we hoped for. It doesn’t mean we can’t get there though. More research and development is required.